See Part I here.
The second part of this cluster of blog posts is one the first of the duo of talks presented by Lynne Boddy. Lynne is a well-known mycologist and researcher and thus, as regards wood-decay fungi, is a good authority from which we can all learn a substantial amount. For a fungi enthusiast such as myself, learning about fungi from one of the best is, in and of itself, very exciting. However, the information presented was equally as exciting, which I shall run through below.
As a slight aside, please watch out for her new book, which is currently being written and should be finished later this year, currently entitled Fungi in Trees. This book will be aimed at the arboriculturist. Lynne is also planning to re-write Fungal Decomposition of Wood, which was a magnum opus co-authored with Alan Rayner.
Fungi invading trees
Primarily, we must accept one core tenet of wood decay: the anatomy of wood has a massive impact upon mycelial networks that sojourn through the wood substrate and selectively metabolise woody cells and their deposits as they go. Indeed, in an ideal world, fungi would gun right for the ray parenchyma, which are incredibly nutritious living cells. However, these cells are very challenging to get to, by virtue of their ‘aliveness’ – living cells in good condition are not easily devoured. Further to this, the sapwood also offers a great for invading fungi, though again – because of the high moisture condition meaning the environment is largely anaerobic (fungi are aerobes and require oxygen to metabolise) – this part of the wood structure is not easily accessed. Of course, the vascular wilts have a better time invading sapwood that is functional, though many fungi will have to bide their time or arrive opportunistically onto and into dysfunctional sapwood if they are to have any means of success in acquiring the treasures within. Thus, is recognising that such living (i.e. conductive and functional) areas of wood are likely just beyond the reach of many fungi, wood-decayers will typically resort to the back-up food source: non-functional xylem vessels within the heartwood or ripewood.
The heart rotters
Now, in accepting this, we come on to perhaps the broadest cohort of wood-decay fungi in living and standing trees (fallen trees have their dysfunctional sapwood metabolised like ravens engulfing a fresh meal) we know of: the heart rotters. These fungi will enter the central wood (i.e. ‘the heart’) through exposed areas of this central column by either sufficiently deep root, stem or branch injury – ultimately, there generally needs to be a continuity of viable ‘heart’ substrate, if any significant degree of colonisation is to take place. Where continuity doesn’t exist, or the fungus finds itself limited to only a certain area, the only manner in which is will typically be able to continue existing is by (1) exiting and finding another host or (2) biding its time and waiting for currently functional sapwood to become incorporated into the heartwood or ripewood, in which it can then spread into – assuming the tree doesn’t lay down defensive barriers that cannot be breached, in order to protect its sapwood, which is in itself a pursuit undertaken to safeguard and hence sustain the high-moisture content of its sapwood (contrary to Shigo’s model, which infers compartmentalisation is largely there with the end in mind of prohibiting fungal succession into the wood). When we look at the ripewood of beech (Fagus sylvatica), we can observe this phenomenon very well – a rosy-coloured ripewood (red heart) with lots of separation lines between instances of decay successions. Ganoderma australe, Ganoderma pfeifferi and Ganoderma resinaceum will afford the most acutely observable examples of this, given their ability to breach such zones by metabolising the phenolic deposits laid down by the tree (this touches upon the idea of a fifth wall in the CODIT model, which will be discussed more later on).
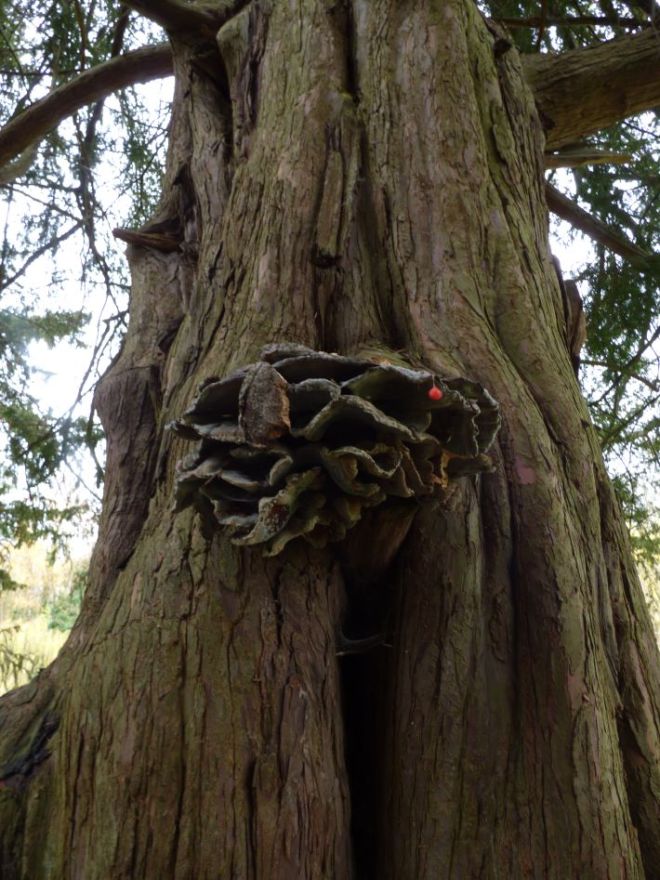
By virtue of the heartwood or ripewood being the least inhospitable (heartwood, in particular, is still often disgustingly harsh, as regards its environment), we can observe immediately some species-specific associations. For instance, the dyer’s mazegill (Phaeolus schweinitzii) will frequent gymnospermous hosts, such as the cedars (Cedrus spp.) and pines (Pinus spp.), whilst chicken of the woods (Laetiporus spp. – often L. sulphureus, though by all means not always, as we will see) will be found often on oak (Quercus spp.), sweet chestnut (Castanea sativa) and yew (Taxus baccata) – it can, indeed, be found on other hosts, as well. On the face value of things, these three tree species have seemingly little in common, though all three have extractive-rich heartwood that this genus can metabolise effectively. In terms of why the genus is referred to and not the exact species, Lynne is of the stance that what we term ‘chicken of the woods’ and default to as L. sulphureus is actually a variety of different species each with their own specialisations – perhaps even down to a specific host tree species (notably for yew, where the chicken species is most pertinently viewed as being a different one).
From a tree management perspective, Lynne then addressed the importance of the heart rotters – what is their impact? Put simply, they change the way in which we view the tree from a safety perspective; as in, when fruiting bodies of heart rotters are identified, if the tree is standing and there exists a target, management considerations are routinely entertained and sometimes the tree is felled. Additionally to this, however, we have other things to appreciate:
- heart rotters do a great job at recycling nutrients, which can then be re-assimilated by the tree when they are uptaken back through its roots (including adventitious aerial roots) or the mycorrhizal fungi the roots associate with
- the wood qualities produced by heart rotters are ideal as habitat for saproxylic insects and nesting birds
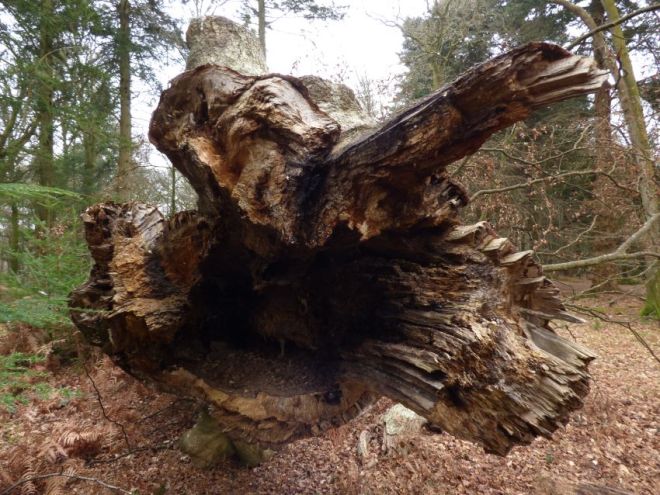
Latent colonisers
Considered the specialised opportunists, such fungi are present within the sapwood or bark, as propagules (thick-walled resting spores known as chlamydospores). Biding their time until conditions are right, wherein the sapwood becomes dysfunctional through means such as wounding or drought (causing embolism), they are perhaps most acutely observed in the years after drought years where they can trigger the formation of strip cankers and resultant reaction growth by the tree (see the below photo). Thus, this year, in the UK, is one to watch out for, as regards such fungi (it might also explain why Kretzschmaria deusta was so abundant this year, given Ascomycetes love dry conditions, which prevailed last summer).

At this point, a delegate enquired as to whether Massaria disease of plane (Splanchnonema platani), as an Ascomycete, could be prevailing in urban conditions recently, because of dry conditions (such as London, where it was been very severe these past few years but prior to that un-noticed). Discussions continued and Frank Rinn interjected to add his thoughts:
- massaria progresses quickly in dry conditions
- recent dry summers have allowed for massaria to thus progress very rapidly (killing branches in as little as three months)
- the lowering of water tables in cities for the construction of basement levels of buildings has meant that plane trees can no longer tap into groundwater supplies
- mature plane trees afford the best conditions for massaria; notably lower lateral branches, which are shaded from the rest of the crown and thus may be most prone to stress
- there have been reports since 1903 from Croatia where large plane trees have shed branches and the massaria fungus was termed the “branch-cleaning fungus”
- conditions are collectively ideal for massaria to become prevalent, as they stand
Reverting back to latent fungi, Lynne then mentioned that she considers fungi to be latent across a broad variety of trees. For example, the coal fungus (Daldinia concentrica), whilst found most often on ash (Fraxinus excelsior) can be isolated from the sapwood of a great range of different broadleaved tree species in the UK. It is, indeed, only when specific conditions arise that are preferable for this fungus that it begins to create mycelial networks – such conditions might not arise in particular trees, or may arise only after conditions suitable for other fungi have arisen and thus D. concentrica then has no capacity to colonise the substrate. Hence, ash remains the core host of this species, in the current climate. However, for the jelly ear fungus (Auricularia auricula-judae), which is also a latent fungus within the vascular system, having been found largely solely on elder (Sambucus nigra) in the 1950s, it is now found on over 20 host species – this marks a huge increase in host range, prompted perhaps by changing climatic conditions.
Wall V
The CODIT model, offered to us by Shigo, details four walls – as can be seen here. As mentioned by Frank Rinn, Shigo himself was considering the possibility of a fifth wall, though this never ‘made it into’ the model. However, Lynne argues that there is the potential for a fifth one, which hearkens back to what was discussed above, as regards rot within the heart of beech wood).
Specifically, whilst the barrier zone (fourth wall) is a zone laid down at the time of wounding by the vascular cambium, the dynamic responses by the tree that occur in real-time as fungal decay advances constitutes a distinction from this initial barrier. Indeed, as decay advances, living cells within the heartwood or ripewood (they do exist; though through mechanisms not fully appreciated, but thought to be associated with the rays running radially through the wood), in addition to the functional sapwood, will, in order to protect the sapwood and keeps its high-moisture quality intact, will plug woody cells beyond the current zone of decay with extractives and phenolic compounds – this will occur within the sapwood most often, though may also be able to occur in the heartwood around the regions where pockets of living cells exist. This response resultantly produces incredibly dense zones of wood that afford the tree’s sapwood a means of protection, which it would otherwise lack, assuming the barrier zone (Wall IV) failed to contain fungal decay. Of course, if this fifth wall fails, another will form, and so on and so forth.
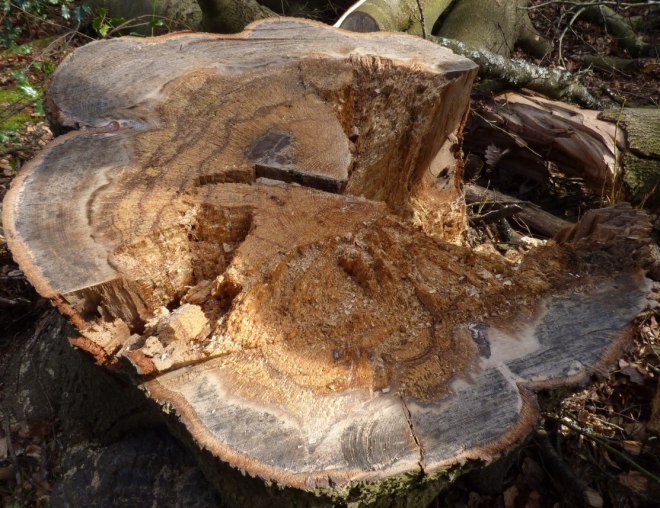
Perhaps we will see this idea discussed more in Lynne’s re-write of Fungal Decomposition of Wood.
Fungal succession
When a tree decays, the fungi that initiated the decay process will not end it. This is because, much like all other ecosystems, as an environment changes those organisms that are best-placed to utilise it change as well. In this sense, fungi are no different – they succeed into a dynamic and ever-altering substrate (wood). Such a phenomenon can be so readily observed when walking into any woodland, when comparing the fungi on standing trees and those in early stages of decay and those much more heavily decomposed. In instances where an entire tree falls and stays largely intact, succession can be most acutely observed, as Lynne detailed with a little help from Ted Green and some research students.
The tree in question, a mature beech, failed and was left, in sections, for ease of its movement into an accessible place, to decay. Along the beech, it was found, through analysis of the wood in the laboratory and by presence of fruiting bodies, different fungi were observed colonising different parts at different stages of decay (as shown below). Such an observation does seem readily apparent, though to have it confirmed through scientific means affords us with an understanding that is more concrete than merely the anecdotal. Indeed, whilst Trametes gibbosa was not isolated from this beech, the presence of Bjerkandera adusta infers that, at some point in the future, T. gibbosa will be found – it parasitises upon the mycelium B. adusta, before then colonising the wood substrate itself. We are, in a sense, therefore, witnessing fungal warfare.

Delving further into the notion of fungal warfare, what is essentially meant is chemical warfare. Fungi synthesise and secrete enzymes, which they use principally to degrade wood, though that can also be used to defend territory or attack other fungi. The result of any fungal battle can be one of four things:
- deadlock, whereby neither fungi gains any ground against the competing fungus
- replacement, whereby one fungus loses its territory entirely by the other
- partial replacement, whereby one fungus loses of some its territory to the other
- mutual replacement, whereby the fungi essentially ‘trade’ places with one another and neither gains any net ground
So how does one determine the outcome of any such skirmish, you ask? Unfortunately, there are so many variables in play that even pitting two fungi against one another in a laboratory is only going to give a slight allusion to what really occurs, though there does nonetheless exist a limited hierarchy of combativeness from which we can assume who the victor will be, under most circumstances (see here at 25:09 timestamp).
Of course, even in assessing this we still have so many caveats to throw in. For example, where moisture conditions are drier because the wood is more exposed, Ascomycetes (i.e. Hypoxylon spp.) will have a better time in securing more wood substrate, as they operate effectively under dry conditions. Indeed, the wood qualities of the substrate itself will even play a role – did the tree uptake pollutants during its life or is it exposed to such pollutants currently, for example. More crucially, if a dead piece of wood (or entire tree) is standing and has thus been subject to relatively dry and exposed conditions suddenly falls to the woodland floor, those fungi reigning when the tree was standing will likely succumb to wood-decay fungi adapted to higher moisture levels and cooler more stable conditions.
The next part of this series will be a brief one on bacteria in wood, as discussed again by Lynne Boddy. I hope to have that written up in the coming few days.